MR contrast agents: A step up from compartmental to targeted imaging
Andrea Martini and Joerg Larsen, of the Institute for Roentgendiagnostics, Braunschweig Teaching Hospitals, Germany, discuss nanotechnology, hybrid imaging and the quest for a personalised medicine.
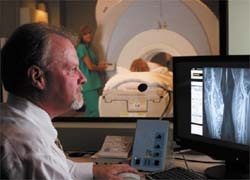
The issue of image contrast is inherent to MRI. The natural desire to differentiate structures and reflect function has previously been explored in Roentgen applications. However, owing to the particulars of ionising radiation physics, k-edge characteristics of only a few agents could be exploited, namely iodinated, Barium sulphate-, Gadolinium- and other heavy metal-based compounds as well as gases. These have been applied to what are essentially compartment-based approaches that may be used to image the vascular system, gastrointestinal tract or other body cavities.
However, in MRI, contrast agent development has been more diverse and can only be considered as a market issue today. With current use quoted for between 20 and 40% of MRI scans, depending on body area, the development of Gadolinium compounds in the 1980s was a particular success story and the largely intravascular use of Gadolinium is dominating current clinical applications for contrast media in MRI. Nonetheless, recent developments somehow follow the evolution of approaches in Nuclear Medicine over two decades ago: Specifically, the attempt to image a physiological environment and pathological processes in vivo is what unites new approaches to modulate image contrast and with the additional advantage of ever increasing spatial resolution. This article aims briefly to review current MRI contrast media usage, the variety of creative approaches to new exogenous media and the state of their development, highlighting particularly interesting or promising concepts.
MRI contrast media were originally used to make unspecific lesion detection easier, i.e. to contrast focal pathological processes, such as inflammation or tumours, against normal tissue background. Lesion characterisation also became possible through repeated scanning following contrast medium administration and was advanced with the introduction of hepatic agents. As hard- and software capabilities improved, fast imaging became a reality and today we routinely image and analyse the first pass of a contrast agent in perfusion studies. Beyond a need for acceptable tolerability, there are no set requirements new agents must fulfil. Quite the contrary, with developments in image acquisition, more specific clinical questions allow for more specific contrast media.
Para- and superparamagnetic properties have traditionally been exploited in MRI contrast agents: Unpaired extranuclear electrons act as strong magnetic dipoles and affect hydrogen nuclei in their vicinity. Gadolinium (Gd) with seven, manganese (Mn) with five and iron with three such unpaired electrons, all shorten both the T1- and T2-values respectively. However, the T1-effect is greater and results in a rise in signal intensity in areas of contrast media uptake on T1-weighted images. Compounds based on these materials have therefore been called positive agents. In contrast, very small particles of iron are too small to be ferromagnetic but they may be magnetised, a state that renders them superparamagnetic. The resulting susceptibility leads to a signal intensity decrease on T2*-weighted images and a negative image contrast, an effect that can exceed that of Gd by well over an order of magnitude [Jensen]. Gd-compounds are available as soluble agents while the ferumoxides come in colloidal solutions (superparamagnetic iron oxide, SPIO).
While Gd-based agents will primarily increase the contrast between normal and pathological tissues when there is an increased vascular permeability, such as in breakdown of the blood-brain-barrier, the site of iron-induced MR relaxation depends on particle size: Larger SPIO will accumulate in the reticuloendothelial system and permit detection and characterisation of focal hepatic or splenic lesions, while smaller particles have a much prolonged intravascular residence and can be used as blood-pool agents. New approaches focus on even smaller, ultra-small USPIOs, enabling cell tracking and the labelling of macromolecules, specifically, antibodies.
In addition, there has been renewed interest in manganese as a cofactor in several critical biological functions where it may serve as a surrogate marker of calcium influx in cerebral or cardiac applications, e.g. to assess tissue viability. Manganese may also bind to particular intracellular sites, giving rise to a unique tissue contrast, which has been used to distinguish neuronal cell layers, e.g. in the amygdala and hippocampus [Koretsky and Silva]. Finally, intracellular manganese can be tracked as it is passed on between neurons and neuronal connections may thus be traced in this way. However, certain states of manganese are toxic and some of the above experiments have been conducted in rodents at doses which are not suitable for the use in humans.
In the late 1990s, a new chapter has opened in MR contrast agent research. Proof of concept was provided for yet another class of agents, however, utilising very different mechanisms: Nanotechnology in targeted or molecular imaging aims to provide specific and sensitive detection of molecular targets that are far too small to be considered with conventional MRI techniques. This approach is analogous to immunohistochemistry, in situ hybridisation, and some scintigraphic methods and positron-emission-tomography [Wickline and Lanza]. While there is considerable experience with issues surrounding the selective binding to target epitopes, the development of agents that provide a sufficient contrast-to-noise ratio to visualise even a single cell constitutes the actual challenge. Nonetheless, this has been realised for some applications in standard clinical MRI settings although mechanisms explored and potential clinical applications are too diverse to attempt to describe the current state of all developments and try to evaluate their future roles.
Nonetheless, a few specific agents from cardiovascular, rheumatologic, neurological, oncological and endocrine research shall be referred to briefly to illustrate the variety of possibilities: Tang has used USPIO particles to detect and characterise the degree of inflammation in atherosclerotic carotid artery plaque, which is today considered the culprit process in plaque instability and subsequent stroke illness. Macrophage activity has been equally assessed in an experimental arthritis to consider MR-based monitoring of disease progression and the effect of therapies [Simon]. Equally, iron oxide accumulation has been shown in spinal nerve roots in a rat model of autoimmune neuritis when uptake interestingly occurred already at a preclinical stage [Stoll]. In a rat-model of transient cardiac ischaemia, French has demonstrated enhancement in hypoxic but later re-perfused muscle using an iron oxide-labelled monoclonal antibody against endothelial cell adhesion molecule PE-CAM 1 at 4.7 Tesla. This could thus serve as a memory agent for ischaemic events. Spuentrup has shown that fibrin clots implicated in acute pulmonary and cardiac thrombo-embolic events can be visualised in swine models at 1.5 T using a prototype Gd-peptide which specifically binds to fibrin. A very high accuracy in focal solid pancreatic lesion detection has been reported for the commercially available Mn-compound Mangafodipir, although the differentiation between inflammatory and neoplastic lesions was poor in that study [Zanello]. Also using Mn-enhancement, beta-cell activation could be visualised in cell culture experiments, perhaps allowing a non-invasive assessment of beta-cell mass and functionality in future [Gimi].
Particular efforts have also been directed at the labelling of stem cells to allow their tracking during therapeutic uses when confirmation of ‘delivery’ and early cell migration are of crucial interest. Iron oxide nanoparticles have been favoured for this purpose, since approaches using radioactive materials have suffered from short-lived tracer activity. Drug delivery may similarly be visualised in this way. A further field looms: the labelling of gene constructs to ascertain the site and expression of certain genes in the context of gene therapy. These developments must be realised in an environment of optimised image acquisition, post-processing and mathematical analysis, a fertile cocktail of opportunities to further exploit biological specificity as outlined by Roberts PL et al. [Eur J Radiol 2000;34:166-].
What evolves from within the mist of this array of new molecular diagnostic agents may be what has been called personalised imaging, the attempt to tailor investigation and therapy to the way a particular illness expresses itself in an individual. However, while embracing these exciting developments, we must not forget that contrast agents are drugs and the fact that it took over 20 years to recognise nephrogenic systemic fibrosis as one of the most harmful side effects of Gadolinium compounds is a sad reminder of this simple truth. Appropriate licensing procedures are therefore mandatory, although the process of development of new contrast media is primarily a technological innovation that leads from the idea of a technical possibility through the conception of a usable compound to finding an application as an imaging tool and market development. This relies on co-operation between academic researchers and industry but priorities on patents may dominate developments as emphasised by de Haen [TMRI 2001;12:221-]. In all this, there is yet another crucial point to consider: As has been pointed out by Rinck, contrast agents require hardware to image them and developments in these markets have not always been synergic [www.emrf.org]. The basic fact that soft tissue contrast and the physical properties of MR contrast agents change and change independent of one another with rising field strengths [Rohrer M et al. Invest Radiol 2005;40:715-] is only the most obvious reason why the career of a novel contrast drug may be unpredictable. Given current bureaucratic obligations in the licensing process and patent-related legal implications, it can take years before a new compound reaches the market and, suddenly, a new hard- or software development comes along and renders the new agent obsolete. We note, for example, that the first work-in-progress PET-MRI-System was presented last year, using next-generation detector technology and featuring acceptable scan parameters such as a spatial resolution of 3 mm, currently sufficient for brain imaging. While the developers and many others primarily see the introduction of further hybrid-modality imaging technology as a commercial development, it will allow new ways to study cerebrovascular and degenerative brain diseases in particular.
Conclusion
New approaches to modulate tissue contrast in MRI are plentiful and diverse and almost all are very much in their developing stages. Evolving methods are faced with a patent-driven market in which only large-scale applications may advance into the latter stages of clinical trials. These include common uses such as occlusive vascular or tumourous diseases. Many invariably cost-ineffective but intriguing concepts in MR contrast media research may therefore require a certain amount of protection or fostering to succeed. Such policies have long been considered for rare medical diseases [Hughes DA et al., Q J Med 2005;98:829-] and may be needed to allow for a small but medically required research and specialist applications market. Irrespectively, the search for contrast in MRI applications is likely to take us to a more facetted picture than may have been imaginable in the early days of the technique.
Full article and reference details: j.larsen@klinikum-braunschweig.de
04.03.2008