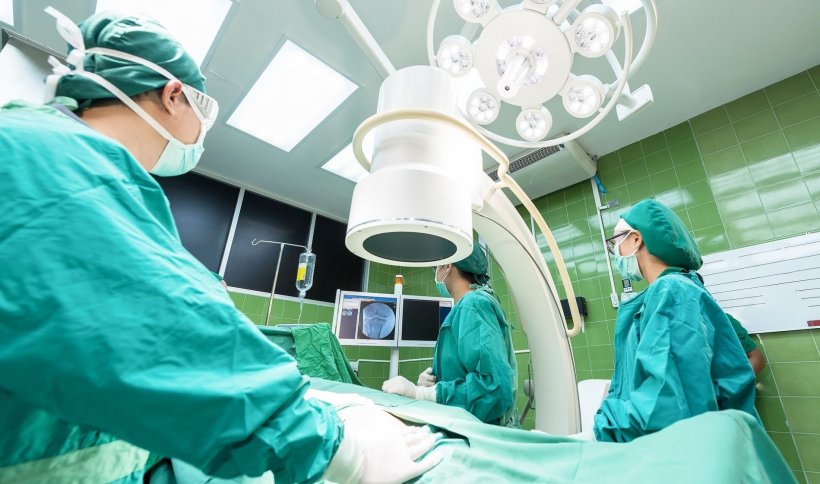
Image source: Pixabay/Sasin Tipchai
News • Microscopy
New imaging technique to improve 3D printed bio-implants
University of Birmingham scientists have developed a new microscopic imaging approach to take a closer look at 3D-printing for developing future patient implants, as well as improved disease modelling and drug screening.
Additive manufacturing (3D printing) platforms create bioprinted structures by moving a special bioink, containing cells, biomolecules and materials, through a narrow tube, but the process can result in cells becoming damaged as they pass through the tiny tube. Using a microscopy technique that shines a blade of light in the material flowing inside the narrow tube, the researchers have been able to examine and reveal important information about how cell damage can occur during the bioprinting process.
The researchers published their findings in the journal Bioprinting.
We used light sheet fluorescence microscopy (LSFM) to mimic the portion of the extrusion bioprinting process in which cells are most likely to be damaged
Gowsihan Poologasundarampillai
The imaging process could give better understanding of bioink flow dynamics and cell movement - enabling investigation of complex capillary designs and process to improve 3D bioprinting and printed constructs. The scientists reveal that their imaging technique illuminates a range of hydrogel-cell behaviour and damage patterns depending on the extrusion speed and properties of the tube used during printing.
Report co-author Dr. Gowsihan Poologasundarampillai, Fellow in Biomaterials and Bioimaging at the University of Birmingham’s School of Dentistry, commented: “Additive manufacturing platforms are transforming research and manufacturing worldwide. We used light sheet fluorescence microscopy (LSFM) to mimic the portion of the extrusion bioprinting process in which cells are most likely to be damaged. We’ve demonstrated the power of LSFM-based imaging to give new insights on cell and fluid movements and flow patterns during extrusion using different bioinks. Previous studies of flow behaviour and mechanical stress on cells during printing lacked this information on cell dynamics, often leading to faulty assumptions about fluid behaviour and flow modeling.”
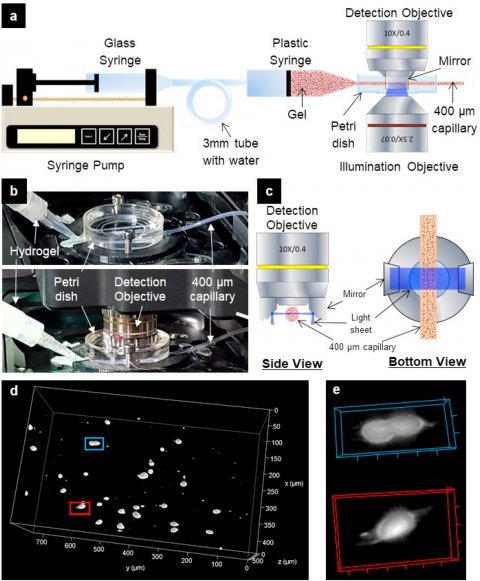
Image source: Poologasundarampillai et al., Bioprinting 2021 (CC BY 4.0)
Bioprinting allows automated structuring of living materials into complex, predefined architectures in a layer-by-layer fashion. These bioprinted structures can then be matured in vitro to generate in-vitro models of tissues and organs for use in disease modelling and drug screening. Extrusion-based 3D bioprinting is used in the biofabrication community due to its high speed of fabrication, precision and simplicity. The process generally delivers a living substance, extruding hydrogel via a thin capillary tube with diameters ranging from 50 μm to 1 mm. “Printing parameters and hydrogel flow behaviour can determine the degree to which cells are mechanically damaged as they pass through the capillary, but direct observation of the hydrogels and suspended cells during the printing process will help shed light on the conditions leading to cell necrosis,” explained Dr. Poologasundarampillai. “We believe that our novel imaging approach could be further exploited to improve the outcomes of 3D bioprinting.”
The researchers applied their imaging technique to study the real-time flow of bioink formulations exhibiting constant and shear thinning viscosities through a capilliary. Despite benefits associated with the extrusion technique, damage to cells can be made worse by the use of smaller diameter capillaries, large flow rates and the high viscosity hydrogels necessary for rapid printing with adequate resolution and shape fidelity. Optimal bioink design involves a careful balance of properties that maximize cell survival and structural stability of the construct.
Source: University of Birmingham
17.05.2021