Image processing - highlighting probabilities and possiblities
Report: Guido Gebhardt
2010 workshop in Aachen, Germany, in March, were welcomed by Professor Thomas M Deserno, head of the Medical Informatics Department at RWTH Aachen University and organiser of what turned out to be an ‘outstanding’ scientific programme.
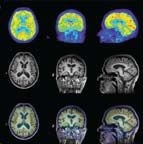
The physician understands the complex physical and chemical processes in the human body; the physicist develops methods to measure and visualise them. Axel Wismüller, of the Rochester Centre for Brain Imaging, University of Rochester, USA, is both physician and physicist.
He and a team of researchers aim to develop novel intuitively intelligible computational visualisation methods for the exploratory analysis of high-dimensional data from biomedical imaging. ‘Specifically, the focus of our research is on developing robust and adaptive systems for computer-aided analysis and visualisation that combine principles and computational strategies inspired by biology with machine learning and image processing/computer vision approaches from electrical engineering and computer science.’
The Centre has a whole body 3-Tesla Siemens MRI scanner and several high-field magnets, and the researchers work at two complementary levels: mathematical algorithms for computational image analysis, and pattern recognition in clinical real-world applications.
Application areas range from fMRI for human brain mapping, MRI mammography for breast cancer diagnosis, image segmentation in multiple sclerosis and Alzheimer’s dementia to multi-modality fusion, biomedical time-series analysis, and quantitative bio-imaging.
When discussing the practical application of segmentation in Aachen, he observed: ‘In medical informatics one is constantly moving between technical feasibility, clinical relevance and practical implementation of new methods.’
One innovation in medical imaging is computer-supported radiology, which automatically segments pathological structures, i.e. vessel and organ delineation are automatically recognised. Although a method not widely used yet, this has great potential. Registration, image sequence analysis and classification are the essential elements of automated segmentation.
Computer-supported image analysis is concerned with multidimensional data. The three spatial coordinates are complemented by data about tissue properties. Automated segmentation methods are considered very promising, particularly for diagnosis and therapy of Alzheimer’s and multiple sclerosis. However, Prof. Wismüller’s team wants to use existing knowledge to monitor the progress and therapy success of many other illnesses in order to apply the segmentation process economically.
The segmentation of the vocal tract, one of his study areas, is a highly interesting application. Researchers synthesise sounds mechanically to support speech training in logopedics. The vocal tract of practised speakers is recorded with MR sequences while phonemes are articulated. The sounds generated by mechanical synthesis clearly conformed audibly to the phonemes. The sound synthesis is based on computational methods that analyse the volume of the vocal tract and determine which sound should be spoken with the respective shape of the vocal tract.
Other applications of segmentation are breast cancer and osteoporosis where the texture of lesions is analysed or the mechanical stability of bones is predicted.
3-T MR BrainPET scanner makes MR-PET possible
A prototype of a new bi-modal scanner has been installed in the Institute of Neuroscience and Medicine, in Jülich, Germany. The scanner combines MRI and PET for brain studies.
The PET detector is located within the bore of the MRI scanner, which makes simultaneous measurements possible. The MR component consists of a commercially available 3-T MRI scanner, whereas the PET detector BrainPET is a new development. The read-out electronics of the PET are based on avalanche photodiodes (APDs) that can be applied in the magnetic field. The inner diameter of the PET ring, consisting of 12 cassettes, is 36cms, so that an MRI head coil can be inserted into the ring.
The first tests were conducted to analyse count rate behaviour and resolution. To assess the image quality of simultaneous MR-PET, phantom studies were performed. The image resolution in the centre was between 2.1 and 2.5m mm in x, y and z directions. First MR-PET studies showed no visible artefacts from the MR image and FDG-images provided high-resolution delineation of the cortex.
Preliminary tests and studies confirm that MR-PET imaging is possible using this newly developed hybrid scanner. Human studies show that the MR images show minimal artefacts, while the BrainPET detector provides excellent image resolution. The PET system saturates at activity concentrations that are higher than those expected in brain studies of glucose or receptor metabolism.
Robust characteristics and segmentation procedures: New approaches
‘Medical image processing is full of unanswered questions. Today, we are at about the same point where optics was 150 years ago,’ said Professor Bernd Jähne, who researches new methods of medical visualisation at the Heidelberg Collaboratory for Image Processing (HCI).
Founded in 2008, within the context of the Excellence Initiative of the Federal Republic of Germany, HCI employs over 80 researchers to identify basic scientific questions in order to solve the problems of the future.
At the HCI, Prof. Jähne and team are involved in basic research, and not concerned with concrete applications. The questions – and answers – they formulate are estimated to become market-relevant in about a decade.
Robust characteristics present an enduring subject. So far, they could only be defined and computed in theory with respect to well-defined, mathematical variances, such as noise, rotation, scaling, affine and perspective depiction. In this case, a specific application would be automated shape recognition -- in the medical context this would be the recognition of tissue structures from various angles and under different light.
ToF (Time of Flight) imaging, one of HCI’s research focuses, has already shown promising results. Similar to an ultrasound system, signal source and receiver are contained in one unit, supplying depth information about tissue. Light is then emitted and the time until the sensor has detected the reflection is measured. A phase shift occurs. Phase shifts and time differences provide an indication of the object’s distance from the source and the surface properties. Colour-coded images are created that supply clear information on the tissue. The aim is to detect tumours better and completely resect potentially malignant tissue.
ToF imaging is a new method with much potential, although there are still some systemic weaknesses. The first systems with only one light source will be ready for commercialisation in a few months. However, it will take a good 10 years until objects can be handled that are also illuminated with ambient light.
With every new segmentation method it is important to obtain a meaningful image with as few clicks as possible. Expert knowledge should be available in seconds, to delineate healthy tissue and tumours in real time. Research focuses on the recognition and identification of boundary layers: Where does a vessel or organ end, and where are the tumour’s boundaries?
17.05.2010