Diffusion weighted whole body MRI
Joerg Larsen MD FRCR and Andrea Martini, of the Clinical Radiology Department, Weende Teaching Hospitals, Gottingen, Germany, describe a new MRI technique that is finding its place in the diagnosis and staging of malignant disease
Malignant diseases rank second in mortality rates in Germany. These patients thus receive a major proportion of ambulant and hospital care, with apparent socioeconomic consequences. To optimise treatment planning, for all solid tumour entities it is mandatory to delineate or stage the primary extent of tumour invasion and spread prior to therapy as precisely as possible.
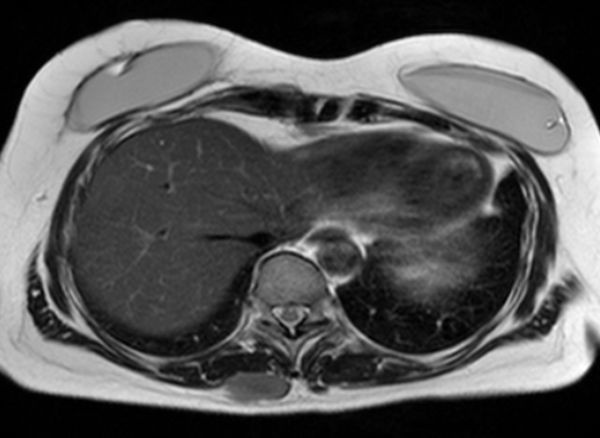
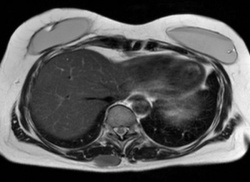
The most widely used staging method is the TNM-system developed by the American Joint Committee on Cancer (AJCC) and the International Union against Cancer (UICC). This classification considers the local advance, metastatic spread into loco regional lymph node stations and distant metastatic deposits. To evaluate the effects of therapy and, specifically, identify recurrent disease as early as possible, follow-up examinations are required.
Computed Tomography (CT)
CT has become the standard technique in diagnostic cross-sectional imaging. Progress in technical developments with helical and sub-millimetre scanning, as well as multi-slice detector systems, are increasingly permitting high temporal and spatial resolution acquisition of volume data. Advances in image reconstruction algorithms as well as contrast media application protocols have followed these developments, adding informative value to data sets. Nonetheless, it must not be forgotten that CT contributes the highest proportion amongst the non-natural causes of ionising radiation to the overall population burden today.
PET-CT
By contrast, positron emission tomography (PET) is a nuclear medical imaging modality that may be combined with CT to improve spatial resolution, i.e. improve allocation of tracer uptake to specific anatomical details in the millimetre-range.
Patients are injected with a radioactive tracer molecule, most commonly 18F-fluordesoxyglucose (FDG). This is taken up by all cells throughout the body, although a hydroxyl group of the glucose molecule has been replaced by the radionuclide 18F. Since FDG-6-phosphate is not metabolised once phosphorylated, it accumulates. The bodily distribution of FDG then permits inference with the glucose metabolism of different tissues. Given that tumour cells tend to consume larger than normal amounts of glucose due to a general increase in metabolism, differential FDG uptake may indicate malignant growth at an early stage. In PET-CT, functional information from PET is thus combined with high spatial resolution CT-data through image fusion.
It is noteworthy that the radiation exposure through a PET-examination alone is of the order of 5 mSv, to which the burden of the CT-scan must be added (approximately 10 mSv for a CT of, e.g. abdominal and pelvic cavities). A particular difficulty arises from the fact that the liver itself is a powerful glucose store, wherefore it physiologically shows a significant uptake of 18F-FDG in the healthy state. The sensitivity of FDP-PET-scanning with respect to focal liver lesions, such as metastases, is thus reduced.
Diffusion Magnetic Resonance Imaging (MRI)
Diffusion MRI is a further imaging method that provides functional information in the area of neoplasia. The process of diffusion principally describes the spontaneous mixing of gases and fluids that are in intimate contact with one another. Diffusion also refers to the equalisation of concentration gradients in solutions.
Diffusion occurs due to thermal motion or, in the case of particles, due to Brownian motion. When measuring diffusion in clinical MRI it is only the extracellular water that contributes to signal intensity. The size of the extracellular space consequently determines image contrast.
MR diffusion imaging using echo-planar imaging techniques has been successfully used in cranial imaging for many years, most commonly to detect restricted diffusion from cytotoxic oedema in areas of ischaemic stroke. However, diffusion imaging is equally helpful when considering other pathologies, specifically solid tumours and epidermoid cysts (increased cellularity), vasogenic oedema (facilitated diffusion), abscess formation (restricted diffusion), haematomas and axonal (neuronal) injury following, for example, cranial trauma.
DWIBS
In the last few years, diffusion imaging has been further developed: most recently the short-TI-inversion-recovery-echo-planar-imaging sequence (STIR-EPI) with a high so-called B-factor has become available, which may be utilised on any body part, even while the patient is breathing freely.
This sequence, termed Diffusion Weighted Whole Body Imaging with Background Body Signal Suppression [DWIBS] (Koninklijke Philips Electronics N.V., Eindhoven, The Netherlands), permits longer scan times than those usually tolerated by patients who need to hold their breath throughout a sequence. Furthermore, a higher than usual number of excitations and signal read outs per slice can be chosen in order to increase the signal-to-noise ratio, which in turn improves overall image quality.
Finally, slice thickness may be reduced to achieve single image volume elements of near equal edge length (isotropic voxels) which allows 3-D post-processing of data sets as in PET-CT.
In contrast to the more commonly used spectral fat suppression technique (SPIR) in diffusion imaging of the brain, fat suppression in DWIBS is achieved through an IR-preparation pulse (STIR), which provides a more homogeneous suppression, specifically in the periphery of the tissue being examined. Through this potent fat suppression, a non-overlapping maximum-intensity projection (MIP) only of restricted diffusion areas becomes possible, enabling the understanding and appreciation of all pathologies at a glance. However, to date, it remains unclear as to why the method functions while the patient is breathing, since it is assumed that motion-probing-gradients that are applied to moving objects don’t produce significant signal.
Therefore, intuitively it does not seem sensible to use such gradients while a patient is breathing, although there are physiological phases of stagnation throughout a normal respiratory cycle and these gradients are being used at very short time intervals of approximately 50 ms (shorter than time to echo). Thus, motion-probing gradients applied during periods of respiratory motion cessation in fact do result in signal return.
Since a number of excitations and read-outs are carried out for each image slice and after signal averaging, this signal achieves meaningful levels throughout a respiratory cycle.
Expectedly, preliminary studies at 3 Tesla suggest that one may exploit the higher field strength to gain signal relative to image noise, albeit at the cost of increased artefacts.
Finally, lesions need to be fully evaluated using ADC-mapping, whereby multiple (≥3) b-values should be used for ADC calculation. For clinical purposes, DWIBS is thus characterised by rapid data acquisition, relative lack of image artefacts as well as the lack of need to administer contrast medium, but does require time for interpretation. In this respect, standards for DWIBS-imaging have still to be agreed upon.
Biological background and research findings
While the exact explanation has not been found for the discovery that diffusion in malignant tumours is restricted, this may be due to a higher cellularity with consequent higher density of diffusion barriers compared with healthy tissues.
Considering data published so far, DWIBS-imaging seems to be highly sensitive for many malignant tumours that return hyperintense signal relative to normal tissues. This is true both for primary tumours as well as lymph node and distant metastases (Images a-d), except for haemorrhagic malignancies and osteoblastic metastases. Destructive and infiltrating bone lesions (e.g. multiple myeloma), lymphoma and gastrointestinal malignancies appear to be particularly well demonstrated. However, specificity has been found to be less than sensitivity, although there may be fewer false-positive findings than with PET-CT. Additionally, there are nonetheless a number of limitations and restrictions that apply to current data: not unexpectedly, artefacts do in fact occur in DWIBS examinations, including movement-, specifically respiratory motion-artefacts, geometric distortions and susceptibility artefacts, e.g. around metallic implants.
Several cohorts of primary tumours, lymph node and distant metastases have been studied to date. These were, in part, rather heterogeneous with respect to tumour entities. Furthermore, most of these samples were relatively small -- between 12 and 200+ cases.
Finally, besides malignant lesions, a number of normal anatomical structures as well as certain benign pathologies were found to be hyperintense on DWIBS in healthy subjects. Importantly, this is not the case with hepatic parenchyma. The liver may thus, in contrast to PET-CT, be well considered by DWIBS imaging (image e).
Outlook - Tumour staging and follow-up play a major role in daily clinical radiology practice. Both are integral to optimised treatment planning and patient management. Commonly, a number of different imaging modalities are utilised in parallel or in sequence: first and foremost the cross-sectional ultrasound techniques, CT as well as MRI and, lately, specifically including the combination of structural imaging with nuclear medicine applications through PET-CT.
Irrespective of the completely different technique, the recently introduced Diffusion Weighted Whole Body Imaging with Background Body Signal Suppression (DWIBS) produces images highly similar to PET-CT-derived appearances and, of course, MRI is rather more available and less costly than PET-CT.
Most of the methods described require a substantial commitment in terms of time and finance. With repeated follow-up examinations, CT also carries a significant radiation burden while the need to administer contrast medium has additional costs, potential side effects and may be obsolete in the case of a previous adverse reaction. DWIBS imaging of pregnant patients and children is of particular interest in this respect.
If diffusion weighted imaging with background body fat suppression proves to be comparable to current gold standards in the delineation of tumour spread, and given their relative limitations, DWIBS is highly likely to become a valuable part of the routine diagnostic repertoire, certainly for some tumour types. Specifically, given that PET-CT has a certain blind spot with respect to liver lesions, the role of DWIBS in staging hepatic spread is of considerable interest.
Contact for article references: larsen@ekweende.de
28.10.2010