Modern radiological diagnostics for the evaluation of muscle diseases
By Erick Amarteifio (1,2), Hans-Ulrich Kauczor (1), and Marc-André Weber (1,2)
Muscular diseases belong to a heterogeneous group with various causes like neurogenic, metabolic, dystrophic, or inflammatory mechanisms as well as channelopathies leading to disorders of the muscle cell membrane potential. In most progressive disease cases the result is a focal or general muscle weakness that, unfortunately, is a very unspecific symptom. Standard neuromuscular literature suggests that radiological imaging would play an inferior role because of absent pathognomonic findings.
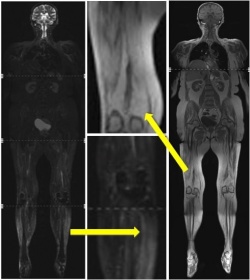
Why does radiologic imaging hold low significance in the case of muscle diseases? Routine magnetic resonance imaging (MRI) protocols will only show morphology and demonstrate oedematous changes, lipomatous changes, atrophy or hypertrophy (fig. 1). Unfortunately, these morphologic changes are not specific. Morphologic changes such as consecutive muscle haematoma after blunt trauma can be shown nicely, but the huge group of myopathies of different origins cannot be differentiated further because the underlying (patho-)physiology is not demonstrated.
The following short review introduces some examples of modern functional imaging methods for evaluation of muscle diseases.
23Na magnetic resonance imaging
Standard MRI bases upon depiction of protons (1H) basically of water and carbohydrate compounds in the human body. 23Na MRI uses the tissue sodium ions (Na+) for image acquisition. A limiting factor is the fact that the emitted signal of Na+ ions is about 20,000 times lower than the emitted signal of 1H. Another limitation is the short T2-relaxation time of Na+ ions, which generates a low signal to noise ratio and thus requires long examination times. Moreover, specific hard- and software is needed, for example a double resonant birdcage coil that can work with the resonance frequency both of sodium cores (16.8 MHz at 1.5 Tesla; 76 MHz at 7 Tesla) and proton cores (63.6 MHz at 1.5 Tesla; 300 MHz at 7 Tesla). This technique has effectively achieved value in the radiologic management of muscle sodium channel diseases.
In the affected patients, an autosomal-dominant bequeathed defect of muscle Na+ channels leads to a pathologic Na+ influx, which causes intermittent or permanent muscle paresis as well as muscle stiffness (Fig. 2A). Typical triggers are exposure to cold or ingestion of potassium-rich food. The diseases appear rarely, examples would be hyper- and hypocalcaemic periodic paralysis or paramyotonia congenita. 23Na MRI is able to depict an intracellular muscle sodium accumulation simultaneous to the development of muscle paresis or muscle stiffness (Fig. 2B). The sodium accumulation correlates well with the grade of paresis and is reproducible. Using special 23Na MRI sequences, a sufficient monitoring of therapy is possible. For example, mexiletine can block pathologic cellular Na+ channels in patients with paramyotonia congenita, which increases the patient’s muscle strength.
With 23Na MRI, a simultaneous decrease of muscle Na+ content becomes visible. Another example is hypocalcaemic periodic paralysis that goes along with intramuscular 23Na accumulation and consecutive muscle oedema. Young patients suffer from muscle oedema, which at a young age can be the only morphologic transformation of their muscle tissue. With proceeding age, a lipomatous degeneration of muscle tissue will take place and the patients develop a permanent muscle weakness, which, however, even then can sufficiently be treated with acetazolamide. The muscle oedema as well as muscle weakness will disappear under treatment, and muscle strength will increase. Recently, the development of 23Na MRI at field strengths of 3 to 7 Tesla (standard are 1.5 Tesla devices) allows for a more precise quantification of intracellular 23Na homeostasis.
31P-magnetic resonance spectroscopy
31P-magnetic resonance spectroscopy was introduced in the 1980s, aiming to evaluate the energy metabolism of muscle tissue. The phosphorous magnetic resonance (MR) spectrum demonstrates several peaks of specific energy metabolites, such as phosphocreatine, which mirrors a typical short-time energy deliverer, as well as inorganic phosphate that is a degradation product. Moreover, adenosine-5´ triphosphate, necessary for muscle contraction, as well as phosphodiester, can be extrapolated. When the chemical shift of inorganic phosphate to phosphocreatine is analysed, the intracellular pH-value can be calculated.
These techniques can successful be used in vivo during muscle exercise and can depict a decrease of phosphocreatine during isometric trial. The technique offers a useful diagnostic tool: For example, in patients suffering from the glycogenosis McArdle`s disease, the muscle glycolysis is disturbed, and the physiological decrease of pH-value due to lactate production does not take place. This can reliably be demonstrated in patients with glycogenosis McArdle’s disease using 31P-MR spectroscopy.
Several research groups tried to find a specific pattern using 31P-MR spectroscopy, but the muscle degeneration finally leads to lipomatous conversion and consecutive loss of high-energy phosphates within the spectrum that can finally be regarded in all chronic myopathies. Moreover, the cross-sectional area of muscle fibres correlates with the amount of phosphocreatine and adenosine-5´ triphosphate. This correlation makes sense, as both metabolites are necessary for muscle contraction. Further developed 31P-MR spectroscopy related imaging techniques allow a spatial illustration of the received phosphocreatine signal and thus a two-dimensional illustration of the muscle energy level.
Proton (1H)-MR spectroscopy
This method has reached a high level of acceptance, because it delivers the opportunity to quantify intramyocellular lipids. Further interesting metabolites are phosphocreatine, choline as marker for rebuilding of the cell membrane, or extramyocellular lipids that are present within the lipomatous intermuscular connection tissue and the subcutis.
However, intramyocellular lipids show a highly inter-individual variability and it is obvious that different physical conditions of muscles obtain different concentration of this lipid fraction. Therefore, it sounds paradoxical that athletes show a high level of intramyocellular lipids and high insulin sensitivity of their muscle tissue. But the intramyocellular lipids serve as energy donators for the muscle mitochondria. Physical work leads to a decrease of intramyocellular lipids. Interestingly, untrained patients suffering from type 2 diabetes with peripheral muscle insulin resistance show a high level of intramyocellular lipids, too.
In the end, many parameters influence the level of intramyocellular lipids, e.g. dietary nutrition, sex, gender, body-mass-index and the oxygen content of tissue. Additionally, modern spectroscopic techniques deliver the possibility of spatially visualising the evaluated resonance peaks with aid of parametric colour maps.
Whole body MRI
Using whole body MRI, the entire human body can be examined within a single examination. It is necessary that the patient has to be completely covered with coils (Fig. 3a). The examination lasts up two about 60 minutes, whereas the duration depends on the protocol and sequence design, for example turbo-spin-echo and gradient echo sequences require comparatively less time than spin-echo sequences. To diagnose muscle disorders, axial and coronal T1-weighted sequences, as well as axial and coronal short-time inversion recovery (STIR) sequences with fat saturation, i.e. suppression, are useful (Fig. 3b).
Contrast media is not needed in most cases. When a biopsy is planned, adequate targets can regularly be found using MRI, e.g. in suspicion of dermato- or polymyositis. The diseases must be diagnosed quickly because, unlike many other muscle diseases, they can be treated successfully with immunosuppressive therapy. Unfortunately, they lead to irreversible muscle weakness when the diagnosis is missed.
A central problem is that muscles may be affected focally, and false negative biopsy findings are found up to 25% in random biopsies instead of image-guidance. In MRI, common findings are oedematous changes of the muscle tissue, which can be identified and mapped using whole body MRI. With the image-guided biopsy, fatty degenerated muscle tissue can nicely be differentiated from oedematous affected muscle tissue as an indication for acute inflammation. However, the pathologic changes of muscle tissue are not very specific. For example, rhabdomyolysis, muscular dystrophy or acute neuromuscular denervation can appear with muscle oedema, too. Generally, oedematous changes give an indication for an acute happening, whereas lipomatous degeneration is observed in chronic stages of disease.
Contrast enhanced ultrasound
Usually, B-mode ultrasound (US) is the first practiced and the most frequently used examination to evaluate muscular disorders. The outcome of sports accidents like ruptures of muscle fibres, haematomas, ruptures of ligaments or extrusion, can well be demonstrated.
In recent years, the development of US made it possible to visualise (patho-) physiological information about muscle microcirculation. Ultrasound contrast media are stabilised microbubbles that stay within the vasculature and thus can be used to evaluate capillary muscle perfusion.
US contrast media of the first generation consist of microbubbles that are burst using high energy US pulses. During the burst, an US signal is emitted, a so called stimulated acoustic emission (SAE), and can be received. Using this technique, increased muscle tissue perfusion in correlation with an increased number of capillaries could be evaluated. During rest, endurance trained athletes obtain an increased muscle tissue perfusion combined with a high density of capillaries compared with non-trained volunteers. Moreover, the quantified local blood volume within the selected US slice correlates with the aerobic capacity and with the perfusion evaluated with venous occlusion plethysmography.
In the case of confirmed dermatomyositis or polymyositis, a significantly higher muscle perfusion compared to healthy volunteers was shown. Additionally, the oedema visible in B-mode US underlines the suspicion of acute myositis. In the case of confirmed polymyositis, immunosuppressive therapy causes increased muscle strength, reduction of creatine kinase level and decreased muscle perfusion at rest.
The first generation US contrast media was disadvantaged in that an evaluation of local perfusion in real-time was not possible. For example, examination during muscular exercise could not be performed. Second generation US contrast media like SonoVue (Bracco Imaging SpA, Milan, Italy) are not destroyed by US waves but are put into oscillation (Fig. 4).
Special US techniques enable the detection of oscillating microbubbles, separation from muscle tissue and deliver the opportunity to assess muscle tissue perfusion in real time. Depending on the grade of isometric physical exercise, a reduced perfusion due to compression of muscle venoles can be observed. At the end of physical exercise an increased US signal could be detected due to reactive hyperperfusion. These findings showed that the resulting hyperperfusion depended on the grade of muscle exercise.
Further techniques
Until now, further interesting techniques to assess muscle physiology and pathophysiology have been developed, e.g. MR- or US-elastography, which enable evaluation of tissue rigidity and differences of tissue elasticity. Elastography is also beneficial to distinguish malignant tissue from normal tissue. With special MR sequences, e.g. diffusion-tensor-imaging, the distribution and integrity of muscle fibre can be evaluated.
In conclusion - Modern US and MR imaging facilitate much more than a simple evaluation of lipomatous, oedematous, hypertrophic or hypotrophic changes within muscle tissue. In addition, muscle microcirculation, sodium homeostasis, energy- and lipid-metabolism, muscle tissue elasticity, and distribution of muscle fibres can be examined.
We expect a much higher level of acceptance for these new techniques in the near future especially with increased knowledge and availability.
1. University Hospital of Heidelberg, Department of Diagnostic and Interventional Radiology, Heidelberg, Germany
2. Department of Radiology, German Cancer Research Centre, Heidelberg, Germany
Contact: Erick Amarteifio MD. Phone: +49-6221-5637265. E-mail: erick.amarteifio@med.uni-heidelberg.de
02.11.2010