Clinical radiologists must embrace their key role in the radiation protection debate
By Joerg Larsen, MD, FRCR, and Johannes Gossner, MD
Most professionals will recognise issues that call for consideration in a wider context, a context that does not merely consider strictly professional issues, i.e. issues based upon facts and figures, write Johannes Gossner MD and Joerg Larsen MD FRCR, of the Institute for Roentgendiagnostics, Braunschweig Teaching Hospitals, Germany.
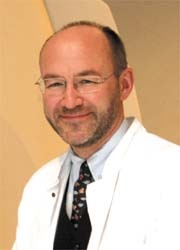
‘These issues have a more general dimension and the current radiation protection debate is such a case: There has been a notable increase in the attention paid to radiation protection issues in the recent past, resulting in a debate that has reached the public domain in the US, perhaps evidenced by the review article of Brenner and Hall in the New England Journal of Medicine last year1. In Europe, radiation protection has long been a ‘on the back burner’, fed by an ever-present subliminal scepticism of scientific advance, a scepticism that, on occasions, has evolved into a diffuse hostility against technical issues, particularly in Germany. The well documented dramatic increase in the number of computerised tomography (CT) scans performed worldwide and the forecasted incidence of up to 2% consequent malignancies, i.e. tumours induced through radiological diagnostic procedures, do however explain the increasing debate only to a degree: the genuinely ‘American’ appearing optimism in scientific advance may have rather suffered a dampening, perhaps in a phase of worldwide tensions, as described by the acclaimed commentator Parag Khanna2, resulting in a discussion that is more focused on associated risks’ Here they review the background and basic considerations in this current, vital debate.
The biological effects of radiation – When any ionising radiation exposes a radiation field, it does what it is supposed to do: it ionises molecules in any material or tissues that lie in its way, whereby some of its energy is absorbed. Depicting this differential absorption that occurs is the basis of the resulting diagnostic image. Consequently, the effects of such ionisations are immediate, even if their biological consequences may not become apparent until years later. Considering the effects on the radiologists of the first hour or on the survivors of the atomic bomb explosions, there can be no doubt that these effects occur. Changes in peptides are largely responsible for these ill effects and occur predominantly through indirect means when free radicals are generated by the ionisation of water, which is ubiquitous in biological tissues. While these tissues have suitable mechanisms in place to repair such damages, these take time and may become exhausted.
To understand risk assessments it is important to be aware of the standard units in which radiation doses are expressed in relation to risks: for each organ exposed, the absorbed dose may be estimated in J/kg [Gy]. Since the effectiveness of ionisation is different for the various types of ionising radiation, the absorbed dose needs to be multiplied by an appropriate weighting factor, resulting in an equivalent dose. This in turn is multiplied by a tissue-weighting factor, which takes account of the different sensitivities of various organs to the effects of ionising radiation. The values for all irradiated organs may be summed up to yield the effective dose to the whole patient. Both the equivalent and effective dose estimates are consequently given in J/kg; however, to signify their biological significance, the unit of Sievert [Sv] has been introduced. This allows comparing the relative risks of different radiological investigations or procedures that utilise ionising radiation.
Furthermore, somatic effects of ionising radiation must be distinguished from hereditary effects: the former occur in the exposed patient, the latter in her or his children. It is noteworthy that, while one would think that such genetic effects should occur, there is actually no such evidence. And yet another piece of information is crucial to understand the whole issue: The somatic effects of ionising radiation can be divided into deterministic ones, which occur once a certain threshold dose is exceeded and statistical effects that are probabilities, i.e. the dose is directly proportional to the effect. Skin reddening through ionising radiation exposure, induction of cataracts, bone marrow suppression and sterility are examples for deterministic effects that in general all require doses that should not occur in a clinical radiology setting. However, given that the lens of the eye has no blood supply, there is no possibility of a repair of damages induced and these will thus be cumulative. In contrast, the induction of leukaemias and solid tumours are considered statistical effects of ionising radiation. These effects may therefore occur at very low doses, while it is believed that they manifest clinically only after a latency of some years (leukaemias), and about 40 years (solid tumours). And whatever the incidence of cancers induced by man-made radiation may be, given that CT was introduced in the 1970s, being then widely applied in the 1980s, we are now within this time range, necessitating the current debate.
Population dose and its development – Ionising radiation is ubiquitous and includes sources of which many of us are not aware: Gamma radiation is emitted from the ground and various building materials just as particularly radon is emitted from the ground, in some locations at significant levels. Equally, our food and drink does contain natural isotopes and high altitude flying brings us nearer to cosmic radiation. Nonetheless, altogether these amount to no more than an estimated average of 4 mSv per annum. Importantly, this includes ionising radiation from medical exposures that contribute about 50% of the population dose. Specifically important is the fact that at least half of the medical exposures are from CT examinations.
Over the past 20 years, the number of CT examinations performed in Western societies has increased by 10 to 20-fold. While other high-dose procedures, such as fluoroscopy-guided interventions, have contributed significantly to this figure, requests for CT of the acute abdomen in particular have risen. Mean doses from CT examinations are of the order of 10–20 mGy but rise to as high as 80 mGy for cardiac applications. Given that many patients do in fact undergo more than one examination during a visit to a CT department, and further, given that surveys have found differences as high as an order of magnitude in applied dose for certain types of examination, with current practice, considerable dose levels are now reached in a large proportion of patients.
However, there are other factors to be considered that contribute to rising dose levels:
• Development of multi-detector row CT machines has both inherently increased dose levels as well as increased the number of indications for CT examinations3.
• There is documented evidence that a significant proportion of radiological examinations are being repeated unnecessarily within time-spans a short as six weeks (audit data, Regional Chamber of Physicians, Lower Saxony, Germany).
• Concerns about litigation not uncommonly give rise to requests for CT examinations, a practice referred to as defensive medicine4.
• Pressures on staffing levels and working hours impinge on sound imaging algorithms.
• Finally, the simple ability to scan has resulted in questionable new indications, particularly in the screening of asymptomatic individuals, where the estimated disease prevalence is thought to be 2% but further investigations result from the examination in as much as a third of cases (whole body CT screening data)4.
Risk estimation and its problems – Hall and Brenner state that ‘there is now direct credible epidemiological evidence of an excess risk of cancer … for these procedures’. This evidence is derived from both the follow-up of the Japanese atomic bomb survivors and the study of Anglo-American nuclear workers published between 1995 and 2007. The mean dose at which an excess relative risk has been identified was found to be 35 mSv, equating to two or three CT scans. The risk of development of a fatal cancer in an individual undergoing such an examination may be estimated by ‘measuring or calculating organ doses, and then applying … specific cancer risk estimates’ (Hall and Brenner). The likeliness of either over or underestimating such a risk has been stated by the US National Council on Radiological Protection to be close to a factor of three both ways. Importantly, the desire to summarise the overall risk of any X-ray examination by using the Sievert as a single effective dose value has been questioned recently6, and a new similarly comparative value suggested (cf. reference). It is noteworthy that the foundations of current risk estimates have been questioned by some, saying that the atomic bomb survivors were exposed to different types of radiation and doses, as are CT patients today. However, considering these estimates, as much as 0.4% of cancers in the US may be iatrogenic from CT use, rising to 2% when its increasing use is taken into account1.
Summary of current thinking on cancer risks – Somatic effects in patients exposed to ionising radiation are a real concern, particularly those statistical effects that occur without a threshold being exceeded. Such doses may result in leukaemias or solid cancers some years or decades after the exposure. In general, this risk is inversely proportional to a patient’s age, i.e. children carry the highest burden of risk. Furthermore, women are principally at higher risk than men due to breast tissue being more radiosensitive during reproductive years. Most importantly, however, the statistical risk for an individual patient remains small with the risk/benefit-ratio in a given clinical setting usually in a patient’s favour.
Approaches to dose reduction – In general, protecting patients, staff and the general public from the ill-effects of ionising radiation may be considered in the context of three sub-headings: justification of any examination (don’t do the test?), optimisation of the actual procedure (applying medical physics) and the limitation of the exposure of employees and the public (Farr and Allisy-Roberts, 1997).
An exposure is justified when the net benefit to the patient is greater than the associated risk. Consequently, repeat examinations when a recent, previous study is not available for review, any screening test, frequent follow-up investigations or studies where the result is inconsequential to patient management, or where a different imaging modality has a higher sensitivity or specificity, must come under particular scrutiny.
Virtually any radiological examination using ionising radiation may be optimised: this refers to the general principle, to keep exposures to a level, as low as reasonable achievable (ALARA) and comprises practical measures regarding both the absolute number of exposures as well as the actual dose applied with each exposure. Either approach requires a thorough understanding of radiation physics, the examination technique and the equipment used. Reference levels have been set for certain types of examinations to address these issues and appropriate quality control measures should be in place as part of a clinical governance system to monitor them.
Finally, dose limits are used to guarantee that staff and the general public are not subject to ill effects of ionising radiation. These are legal limits and staff exceeding certain dose levels may be placed under particular monitoring and certain restrictions.
Special considerations apply to the management of female patients and staff who may be or may become pregnant, as well as to breast-feeding mothers. However, these are beyond the scope of this brief review and readers are referred to previously issued guidance such as from the Royal College of Radiologists of London.
Practical measures to reduce patient dose – A detailed analysis of the dose increases outlined above is key to possible dose reductions: In CT usage, there have been suggestions that up to a third of scans are either unnecessary or may be replaced by alternative imaging as the screening of asymptomatic individuals in the context of lung or colon cancer, cardiac disease and whole body screening, where the benefits have still to be established. The screening of at-risk individuals for abdominal aortic aneurysms is a similar scenario: here ultrasound may be applied to equal effect, instead of the admittedly convenient CT. CT usage may equally be reduced by re-considering other common indications, specifically in the pre-operative assessment of presumed appendicitis, when patients are frequently young and thereby more radiosensitive.
With respect to X-ray technique, there are ample ways of reducing the applied dose per CT scan or Roentgen-examination by adjusting technical factors: It is important to note that any dose reduction (specifically in the milli-amperage) will result in an increase in image noise. A trade-off is thus necessary between acceptable, i.e. diagnostic quality images, and dose. This relation is favoured when there is high, inherent, image contrast (renal stone disease), when only localising information is required (PET-CT) or when small subjects are being scanned (children) who exhibit less secondary radiation scatter, a large factor in image noise. The issue of differences in the absorption of radiation in relation to patient size and organ scanned has been addressed by the introduction of automated i.e. machine recommended exposure control in modern CT scanners. Furthermore, reducing the body area scanned to a bare minimum, or the number of contrast medium-enhanced phases, will have a considerable impact. Shielding techniques include gonad protection and bismuth-coated eye shields. Radiographic dose reduction e.g. includes left- instead of right-sided lumbar spine radiography, beam angulation in cranial CT to exclude the lens of the eye, and copper-filtration as well as the use of pulsed beams in fluoroscopy. Clearly, the measures indicated here do not constitute a comprehensive list but are examples for specific, i.e. targeted, modifications in the examination of individual patients.
Final remarks – Considering all the above, surprisingly few certainties appear to remain. The foundations of current risk estimates have been questioned by some, just as the use of the effective dose concept has been criticised. However, within the agreed range of uncertainty of ± a factor of three, we may concur that there is a notable risk to public health with current practices in the use of CT.
CT examinations are commonly performed in the elderly and seriously ill whose life expectancy is not that of a normal population. Nonetheless, CT use in the young and for screening purposes should be questioned. In addition, we must take care when justifying repeat examinations and when alternative imaging modalities are available. These certainties are at the interface of clinical medicine and clinical radiology; specifically, we may condense them into the questions whether or not, and how, any examination utilising ionising radiation is performed: Radiological expertise and guidance are central to this decision-making process. Radiologists’ unique understanding of radiation physics, examination techniques and the equipment used therein is central to dose reduction in any shape or form.
This conclusion is as important as the understanding that we need to raise awareness of the fact that population exposure is reaching a level that may constitute a public health issue.
It has been argued that further regulations may be required to ascertain standards in radiation protection. We believe that this approach would likely fail, because it could not be meaningfully enforced. In contrast, if clinical radiologists could embrace their central position and practice radiation protection as shining examples, attitudes and practices of referrers and other staff working with ionising radiation may change. Detailed referral criteria and guidelines such as issued by the European Commission (Referral Guidelines for Imaging, www.europa.eu) are prudent adjuncts to this argumentative repertoire when justifying and performing Roentgen-examinations. And, as pointedly quoted by Cohnen recently, ‘in radiation protection, a gram of brain weighs more than a ton of lead’ (paraphrased from Wachsmann, 1965).
References: 1. Brenner DJ and Hall EJ (2007) NEJM 357:2277-84. 2. Khanna P (2008) The Second World: Empires and Influence in the New Global Order. Random House, London. 3. Dawson P (2004) Br J Radiol 77:S10-3. 4. Hall EJ and Brenner DJ (2008) Br J Radiol 81:362-78. 5. Preston DL et al. (2007) Radiat Res 168:1-64. 6. Brenner DJ (2008) Br J Radiol 81:521-3
03.11.2008